Hearing: Sensing the World Through Sound Waves
What is sound?
Physics defines sound as a vibration that spreads though air or water as a mechanical wave of pressure energy. A sound wave may be composed of oscillating gas or oscillating water molecules. Sound spreads through gases and water as compression waves much like the wave form created by the surface tension of water in response to an object dropped into a quiet pool.
Compression waves cause local regions of closely packed and of widely separated molecules. It takes some form of energy, like the kinetic energy of a stone breaking water’s surface tension, to set a sound wave in motion. Each wave scatters its energy as it slowly spreads from its initiation site to distant points.
The distance between successive crests of a wave is its wavelength. Sound waves that can be detected by the human ear possess wavelengths ranging from 17 meters to 17 millimeters, which is 20 Hz to 20,000 Hz.
Hearing sounds
The brain is very good at hearing sounds. But how does a brain identify the object(s) that set sound waves in motion?
Seldom does a sound wave in the environment arrive alone at the ear, the body’s interface for sound. Usually the ear receives a complex mixture of sound waves simultaneously. The brain can segregate and group the regularities in complex patterns of sound waves that the environment provides. The grouped patterns are then compared with its stored information about language and memories of previous sound experiences.
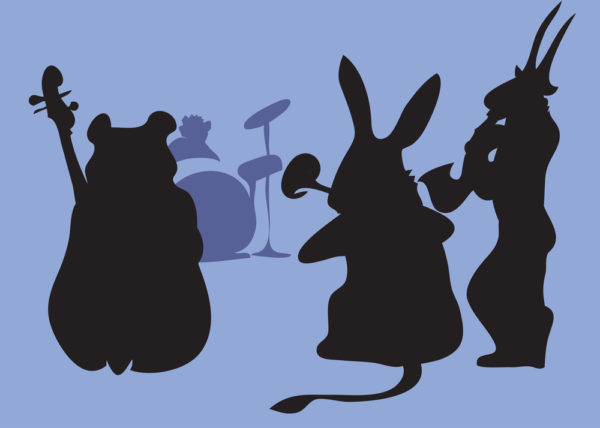
Brain’s ability for sound source separation permits hearing each instrument separately, Ingaga/Shutterstock.com
Physiology and psychology define the basic unit of hearing as the ‘auditory object’. For example, when an orchestra plays, a listener is able to hear and identify each instrument as a separate auditory object: a drum, or a saxophone, or bass.
While attention to sound is not necessary for hearing, it may guide hearing. It is possible, for example, to focus on one part of a mixture of sounds and block out the rest. With careful listening to a group such as is pictured here, a person could choose to listen to the drum and tune out the other music.
Anatomy of the human ear
The anatomy of the human ear is functionally divided into three compartments, the outer ear, the middle ear and the inner ear. Each of the ear’s three compartments plays an essential role in converting mechanical sound waves into electrical signals that the brain can understand.
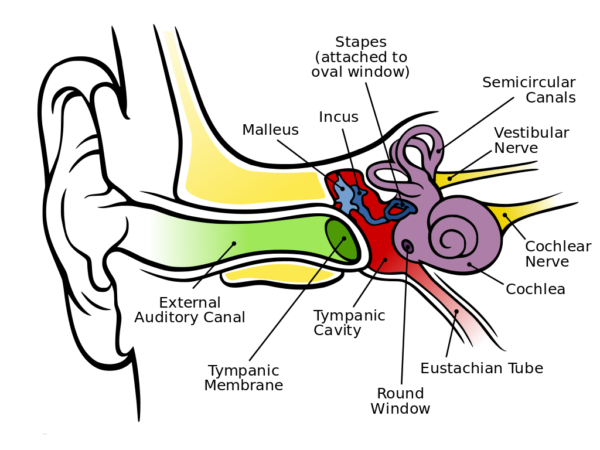
Anatomy of human ear is designed to convert air compression waves into neural signals interpreted by the brain as sound, Chittka L Brockman/Wikimedia Commons
Outer ear
The visible part of the ear is also called the pinna or auricle. It is a skin covered thin plate of elastic cartilage connected to the head by ligaments and muscles. The outer ear includes the pinna and the external auditory canal that funnel sound waves to the tympanic membrane at the canal’s end.
The shape of the pinna sorts sound waves selecting for those in the frequency range of human speech. The external auditory canal selects for sound waves moving in a specific direction. Have you ever turned your head to listen better for a sound? Sound waves reaching the end of the canal transfer their vibration energy to the tympanic membrane.
Middle ear
The middle ear is the hollow chamber between the outer ear and the inner ear. It is connected to the pharynx by a narrow tube called a Eustachian tube. This connection permits equalization of pressure on both sides of the tympanic membrane.
The middle ear contains three small bones with the general name of ossicles. Their specific names malleus, incus and stapes describe their shapes as hammer, anvil and stirrup. They form a coupling device that transfers the vibrational energy of the tympanic membrane to the fluid-filled inner ear, the cochlea.
The tympanic membrane, also known as the eardrum, is fused to the malleus, which connects to the incus, which in turn connects to the stapes. Vibrations of the stapes footplate at the oval window of the cochlea introduce pressure waves in the cochlea’s fluid-filled chambers.
Inner ear
The name of the inner ear, the cochlea comes from a Greek word meaning spiral and describes its shape. The cochlea is a hollow tube divided lengthwise into three fluid-filled canals.
The outer two canals, the scala tympani and scala vestibuli, begin at the oval window and the round window at the base of the spiral. The third canal is sandwiched between the outer two canals and is called the scala media. The scala media contains a structure named the Organ of Corti.
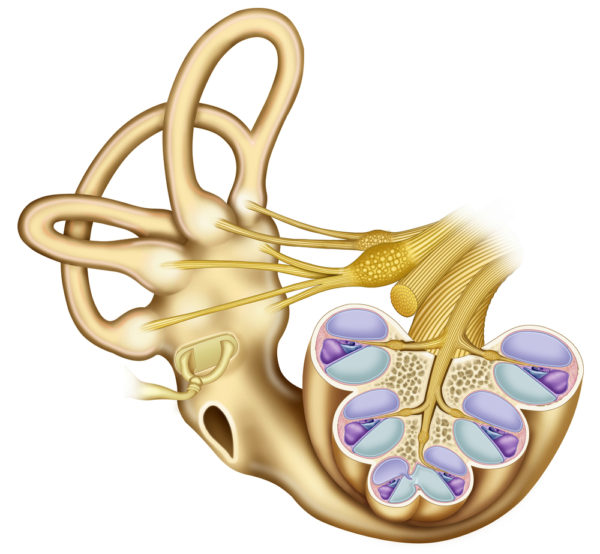
Cross section of the cochlea spiral showing the Organ of Corti sandwiched between the outer two canals, Alexilusmedica/Shutterstock.com
Cells within the Organ of Corti sense the fluid motion created within the two outer canals by vibration of the stapes. Sound wave sensing cells of the Organ of Corti get their name from the tufts of cilia that protrude from their apical surface. They are called hair cells. There are two distinctly different types of hair cells in the Organ of Corti, outer hair cells and inner hair cells.
The tallest cilia of the outer hair cells make direct contact with, and are thought to be connected to, the tectorial membrane positioned above them. The inner hair cells connect with the tectorial membrane through motion of the fluids.
Inner hair cells transform vibrations in the cochlear fluids into electrical signals by opening and closing ion channels at the tips of the hair bundles. This sets in motion a sequence of events that causes release of neurotransmitter at the cell’s base.
The neurotransmitter in turn induces action potentials in the cochlear neurons which contribute their axons to the VIII cranial nerve to the brain. The cochlear neuron pathway terminates at the auditory cortex of the brain. Each inner hair cell has numerous auditory neurons at its base.
Outer hair cells are only found in mammals. Neurons at the base of the outer hair cells deliver signals from brain processing regions that modify the cells’ response to fluid waves. Outer hair cells mechanically amplify low level sounds more than loud sounds and reduce a wide range of sound pressures to a small range of hair displacement. Bending of the stereocilia on an outer hair cell produces an influx of K+ into the cell through ion channels. This change in the internal electrical field leads to shortening of contractile fibers within the cell changing its shape.
The rigidity of the basilar membrane on which the hair cells sit varies along the length of the cochlear spiral. The stiffness arises in part because the thickness and the width of the basilar membrane are not uniform. The most inflexible section of the basilar membrane is near the oval window where the stapes initiates motion in the cochlear fluid. At this area only high frequency vibrations move the membrane and therefore the hair cells.
Closer to the cochlear apex the basilar membrane is much more flexible. When low frequency vibrations are transmitted by the stapes to oval window the stiffer membrane near the window fails to move, but fluid waves reaching the apex easily re-position the distal section of the basilar membrane. The net effect is that high frequency sound is sensed by hair cells near the oval window and low frequency sounds by those at the apex of the cochlea.
Auditory cortex
The axons of the neurons positioned at the base of inner hair cells form the auditory nerve which joins the axons of the neurons originating at the other part of the inner ear, the vestibular apparatus or semicircular canals, to form cranial nerve VIII into the brain. The auditory nerve pathway through the brain travels to signal-processing areas of the thalamus on its way to the section of the cerebral cortex devoted to auditory analysis, the auditory cortex.
The primary auditory nerve pathway from the inner ear to the auditory cortex is supplemented by other neuron pathways from several areas of the brain. Supplemental pathways that influence hearing travel to the auditory cortex and to the base of the outer hair cells. Brain areas included in auditory fine-tuning are those involved in attention, remembering and emotion. They include cerebral cortical areas allotted to decision making and working memory and also deeper brain areas dedicated to emotion.
Vestibular apparatus
I want to include something about the vestibular apparatus even though its job is not hearing. The inner ear is encased in the bone of the skull and was missed by early anatomists. It was discovered at the beginning of the 20th century because it also contains the balance apparatus that senses position of the head.
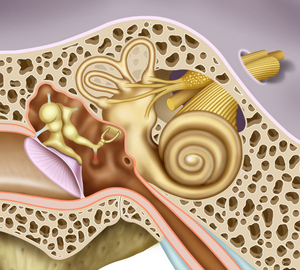
Vestibular apparatus of the inner ear is completely encased in the bone of the skull, Alexilusmedical/Shutterstock.com
Presence of the inner ear in bone was first predicted by an ear doctor named Robert Barany. He treated patients for ear wax by syringing their ear’s external auditory canal with water.
Barany used ambient temperature water to do this, and his water was usually cold. This procedure made his patients quite dizzy. One patient told Dr. Barany that he did not think he would get dizzy if the doctor used warm water instead of cold water.
In trying to accommodate his patient, the doctor used hot water the next time he syringed this patient’s ear. Well that did not work well either. The patient still became dizzy.
The patient told him ‘Not hot water, warm water!’ or something to that effect. When the doctor tried syringing the ear again with water at body temperature, he discovered the patient was correct – no dizziness.
This patient encounter led Dr. Barany to ask three questions. First, what properties would a physiologic system need to explain his patient’s response? He decided that it must be a fluid containing system. Second, what shape would such a system have to assume? He predicted the shape of the semicircular canals. Third, where is the system located that it escapes detection? Near the ear – encased in bone.
Dr. Barany’s subsequent investigation led to discovery of the bone-encased inner ear and the semicircular canals of the vestibular apparatus. For his ability to move from a simple observation to a theory and then to discovery, Dr. Barany received the Nobel Prize in Physiology and Medicine in 1914.
A video by 2 Minute Neuroscience provides a description of the vestibular apparatus that will show another use of hair cells in the inner ear. The hair cells of the vestibular apparatus are analogous to the inner hair cells of the cochlea of the Organ of Corti, although the overlying membrane is different.
Further reading:
How the Human Brain is Organized
Neurons: Where Does Their Electricity Come From?
Do you have questions?
Please put your questions in the comment box or send them to me by email at DrReece@MedicalScienceNavigator.com. I read and reply to all comments and email.
If you find this article helpful share it with your fellow students or send it to your favorite social media site by clicking on one of the buttons below.
Margaret Thompson Reece PhD, physiologist, former Senior Scientist and Laboratory Director at academic medical centers in California, New York and Massachusetts is now Manager at Reece Biomedical Consulting LLC.
She taught physiology for over 30 years to undergraduate and graduate students, at two- and four-year colleges, in the classroom and in the research laboratory. Her books “Physiology: Custom-Designed Chemistry”, “Inside the Closed World of the Brain”, and her online course “30-Day Challenge: Craft Your Plan for Learning Physiology”, and “Busy Student’s Anatomy & Physiology Study Journal” are created for those planning a career in healthcare. More about her books is available at https://www.amazon.com/author/margaretreece. You may contact Dr. Reece at DrReece@MedicalScienceNavigator.com, or on LinkedIn.
Dr. Reece offers a free 30 minute “how-to-get-started” phone conference to students struggling with human anatomy and physiology. Schedule an appointment by email at DrReece@MedicalScienceNavigator.com.
Comments
Hearing: Sensing the World Through Sound Waves — No Comments
HTML tags allowed in your comment: <a href="" title=""> <abbr title=""> <acronym title=""> <b> <blockquote cite=""> <cite> <code> <del datetime=""> <em> <i> <q cite=""> <s> <strike> <strong>