Ion Channels
Ion channel selectivity
Ion channel selectivity is important to neuron signaling, muscle contraction, kidney function and much of the rest of physiology. An ion, an electrically charged atom, can only travel through cell membrane channels designed specifically for it. Selective ion channels are found throughout the body. No matter which body system you study, membrane ion channels will play some role in its physiology.
Physiologic processes rely upon which ion channels in a cell’s membrane are open and which are closed. This article focuses on describing two types of plasma membrane channels, those that recognize the sodium ion [Na+] and and those that service potassium ion [K+].
Ion channel filter
Biologic membranes are composed of several types of fat (lipid) molecules, labeled number 6 in this illustration. Water soluble particles such as electrically charged ions do not pass through lipid membranes without help. A channel for ions, an opening that traverses the membrane, is needed. For ion flow through the channel to happen, the channel must be open to the fluids on both sides of the membrane.
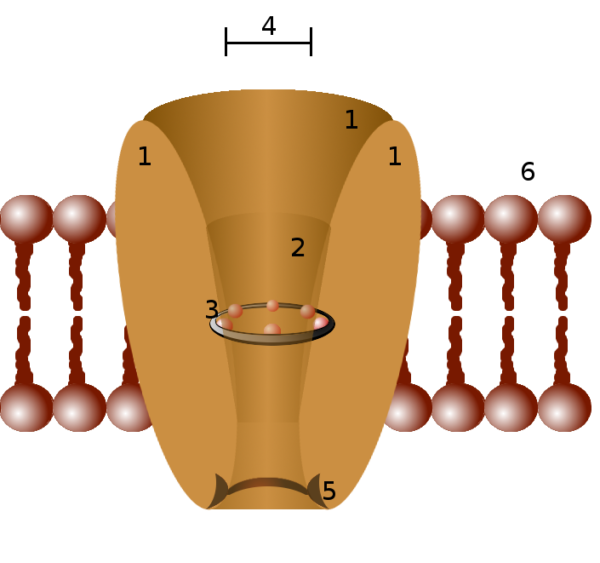
Parts of an ion channel protein, #3 represents the selectivity filter, Pawel Tokarz/Wikimedia Commons
The interior of an ion channel must be able to attract its ion. That is, it must be lined with molecules that carry an electrical charge that is opposite the ion’s charge. Also, the channel core must be large enough for one type of ion to pass, but small enough to block the passage of other ions.
Membrane ion channels consist of proteins that assemble themselves in various configurations as they fit themselves into a cell membrane. Somewhere within each ion channel protein there is a selectivity filter, labeled number 3 in the figure above. The ion selectivity filter allows passage of only one type of ion.
Ion channel proteins
Proteins have characteristics that make them especially good for working as ion channels. First, ion channel proteins form spirals called helices. Lipid-soluble amino acid side chains to the outside of the helix fix ion channel proteins into cell membranes. Amino acid side chains lining the interior of an ion channel composed of several helices have an electrical charge to attract ions. The portions of ion channel proteins that traverse the cell membrane are in the helical form shown here.
Second, ion channel proteins change their shape readily when they encounter fluctuations in the electrical field surrounding them or when a chemical binds to them. Their ability to change their shape allows the ion channel’s selectivity filter to open and close. Opening and closing of ion channels is a major form of cellular communication, it is the basis of neuron action potentials and synaptic transmission.
Potassium ion channel
Two very important ion channels found in the body, often near each other on the cell membrane, are the potassium ion channel for K+ and the sodium ion channel for Na+. The molecular weight of Na+ is substantially less than that of K+. Both ions carry a single positive charge, and the space occupied by a Na+ ion is less than that occupied by a K+ ion.
So, why does smaller Na+ not pass through the selectivity filter of the potassium ion channel? It is only recently that scientists solved this mystery. The K+ channel is an excellent example of how a protein channel can be ion selective.
Ions in water solution are surrounded and bound loosely by water molecules. This arrangement is called a hydration sphere. An ion hydration sphere is much too large to fit into the passage of an ion channel. So first the water must be displaced. To strip water molecules away, an ion must meet another molecule with which it can form a bond that is stronger than its hydrogen bond with water.
Structural analysis of K+ ion channels from a variety of species demonstrated that they are lined with carbonyl oxygen. Carbonyl oxygen, oxygen double bonded to carbon, can be found in the carbon backbone structure of all proteins. The position in space of a protein’s carbonyl oxygen varies depending upon how the protein is folded in upon itself. In the potassium channel the carbonyl oxygen, with its partial negative charge, is in exactly the correct position to compete with water molecules for K+ at the entrance to channel.
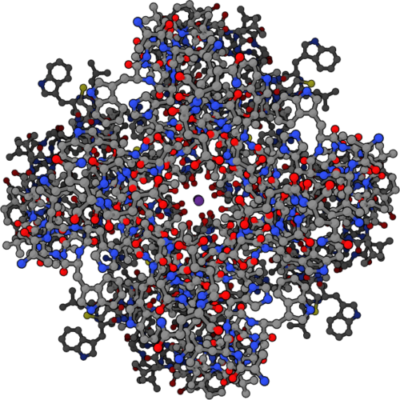
Four part symmetrical structure of potassium ion channel with potassium ion at center, Public Domain/Wikimedia Commons
The potassium channel illustration above shows the entrance to the potassium channel looking down from the top with a single K+ (purple dot) inside. Notice the red oxygen molecules surrounding the purple dot. This illustration is based upon data for this channel in the Protein Data Bank, file number 1BL8.
The same process does not work for Na+ at the K+ channel, because Na+ ion is smaller than K+ ion. The carbonyl oxygen moieties lining the K+ channel opening are too far apart to form a competitive bond with the smaller Na+ ion, leaving the hydration sphere in place. With its hydration sphere intact, Na+ is much larger than a bare K+ – too large enter the potassium ion channel.
Sodium ion channel
As you probably guessed, the sodium ion channel has an entirely different configuration than the potassium ion channel because it uses different protein features to achieve its selectivity.
Na+ channels consist of one large protein, the alpha subunit, that works with 2-3 smaller proteins. The large alpha subunit protein is spread out in a cell membrane for illustration in the image below. The large alpha subunit contains the ion conducting channel when it is in its normal membrane position.
The sodium ion channel is regulated by the presence of one or more of the three smaller proteins. Each of the smaller proteins is about 10-15% of the size of the large protein. Presence of the smaller proteins sometimes alters the cellular location of the larger protein in the membrane and on occasion alters its sensitivity to the electrical field of the membrane.
The diagram presented above illustrates the sections of the large protein of a sodium ion channel. In this diagram, N is the beginning of the protein’s amino acid sequence and C is the end of the sequence. Notice that both ends are inside the cell. G is where sugars are added to the extracellular portion of the protein. P are the sites where the protein can have phosphate groups added. S is the location of the ion channel components. I indicates the sites affected when the channel is inactivated blocking entry of Na+. The four sets of transmembrane helices are each labeled 1-6.
In vivo the four sections, labeled I-IV in the picture, form a circular cluster in the cell membrane. Ion selectivity for the Na+ channel is created by the protein loops between the helices labeled 5 and 6 in each of the four sections. Notice the protein loops between helices 5 and 6 are on the outside of the membrane.
As the four sections of the channel group themselves together in the membrane, the four large loops between transmembrane helices 5 and 6 interact with each other to create the selectivity filter for Na+ ion. As with K+ at the potassium ion channel, water is stripped from Na+ by its electrical interaction with its channel filter. The area between helices 5 and 6 labeled S are open to the inside of the cell.
The Na+ and K+ ion channels of the neuron axon are sensitive to electrical changes in the cell membrane and are named voltage-gated ion channels. The Na+ and K+ ion channels on neuron dendrites open in response to chemicals and are named neurotransmitter channels. Ion channels and ion channel selectivity is still an active area of physiology research. For example, scientists are still trying to measure whether any of the displaced water molecules of the hydration spheres follow the ions through ion channel proteins.
Do you have questions?
Do you have questions about the physiology of ion channels? Please put your questions in the comment box or send me an email at DrReece@MedicalScienceNavigator.com. I read and reply to all comments and email.
If you find this discussion of ion channels useful, please share it with your fellow students or send it to your favorite social media by clicking one of the buttons below.
Further reading
Water’s Chemistry Governs Physiology
Physiology: Custom-Designed Chemistry
Margaret Thompson Reece PhD, physiologist, former Senior Scientist and Laboratory Director at academic medical centers in California, New York and Massachusetts is now Manager at Reece Biomedical Consulting LLC.
She taught physiology for over 30 years to undergraduate and graduate students, at two- and four-year colleges, in the classroom and in the research laboratory. Her books “Physiology: Custom-Designed Chemistry”, “Inside the Closed World of the Brain”, and her online course “30-Day Challenge: Craft Your Plan for Learning Physiology”, and “Busy Student’s Anatomy & Physiology Study Journal” are created for those planning a career in healthcare. More about her books is available at https://www.amazon.com/author/margaretreece. You may contact Dr. Reece at DrReece@MedicalScienceNavigator.com, or on LinkedIn.
Dr. Reece offers a free 30 minute “how-to-get-started” phone conference to students struggling with human anatomy and physiology. Schedule an appointment by email at DrReece@MedicalScienceNavigator.com.
Comments
Ion Channels — No Comments
HTML tags allowed in your comment: <a href="" title=""> <abbr title=""> <acronym title=""> <b> <blockquote cite=""> <cite> <code> <del datetime=""> <em> <i> <q cite=""> <s> <strike> <strong>