Physiology: Why Learn Its Chemistry Now
Avoid struggling with the chemistry of physiology
Do you struggle with explanations of the chemistry used to describe physiology? Do find molecular processes like ion channels, osmotic pressure, and hydrostatic pressure difficult to master?
What about all those metabolic pathways you memorize? What is that mysterious stuff called energy that is carried around by the ATP molecule?
Do you think there must be some way to memorize the physiology of each system? Are you frustrated in trying to figure it out?
There must be some way to make learning physiology easier, but what is the secret! Surely, there is a formula for learning human physiology.
A formula for learning physiology
Because a few simple laws of chemistry and physics are used over and over in every body compartment to explain its function, the formula for learning physiology begins with learning a little chemistry. Physiologic chemistry is a customized subset of chemistry’s laws because the reactions take place in a water solution, within a narrow temperature range, and at a specified pH.
In contrast, organic laboratory chemistry includes a broader set of rules to accommodate an array of fluid solvents, temperatures, and pH values for rearrangement of biologic molecules. You do not need a full course in organic chemistry to understand the molecular processes of human physiology.
Basic concepts of physiologic chemistry
Chemistry’s definition of energy
Chemistry defines energy as the ability of atoms and molecules to move through space. Experiments demonstrate that atoms and molecules, which are groups of atoms linked together, travel through space and therefore possess kinetic energy. The energy of small gas molecules like oxygen when stored in tanks can be measured by pressure gauges.
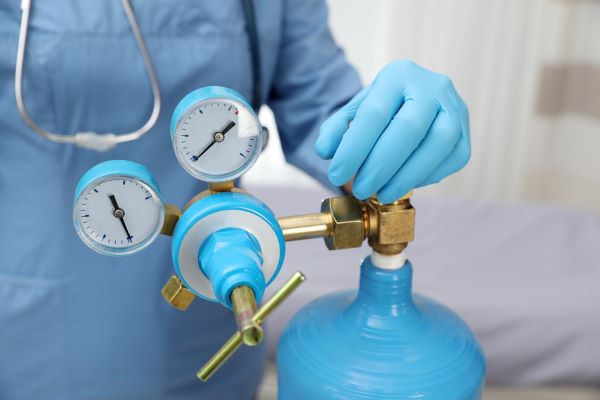
The amount of pressure recorded by gas gauge signifies the quantity of gas in the tank. New Africa, Shutterstock.com
But large molecules also energetically move. How much molecules move depends upon the temperature of their environment. The higher the temperature, the faster atoms and molecules move through space and the greater the energy they possess.
Maintaining human body temperature at a set point of about 98 degrees Fahrenheit limits the energy of physiologic molecules. That is, controlling the range of body temperature restricts the kinetic energy of the body’s molecules, protecting structural and functional proteins and lipids.
Atoms that can physically bond to each other by sharing electrons will do so when they come close enough to each other in the correct orientation. When atoms bond to each other, the bond restricts some of their movement, their kinetic energy.
The stronger the bond that forms, the greater is the decrease in kinetic energy of the participating atoms. But the atom’s energy does not disappear from the system. When the bond is eliminated, the kinetic energy of the atoms is either released to the environment as heat, or it is incorporated into another molecule.
Bonds between atoms vary in their strength. Therefore, the amount of kinetic energy that is released when the bond is broken also varies. Strong, low energy bonds contain a great deal of suppressed kinetic energy. Weak high energy bonds contain a relatively small amount of suppressed kinetic energy. Keep this in mind when you read about ‘high energy molecules’ in metabolic pathways named ATP.
Another kind of molecular bond also plays a role in living systems. It is called a hydrogen bond. The water molecule that forms the solvent system of the body forms hydrogen bonds with itself and with other biologic molecules like proteins.
Water chemistry, hydrogen bonds and pH
Water is a is composed of small high energy molecules. Each water molecule is in constant motion at body temperature, forming-breaking-reforming hydrogen bonds with other water molecules.
Water molecules are polar, have a negative and two positive surfaces. These surface charges are partial charges, less than equal to the strength of an electron. A weak type of bond called a hydrogen bond forms between the partial charges of the water molecule and the charged surface of ions and other large molecules like proteins.
Water molecules fail to hydrogen bond with the uncharged surfaces of fats and lipids. This fortunate situation leads to the formation of lipid cell membranes and, therefore, cells and tissues.
Another important property of water is its tendency to break apart into a single hydrogen ion and a hydroxide ion. Only a small portion of a pure liquid water solution does this. How many ions form in pure water has been measured and that quantity was assigned the value of pH 7.
- H2O → [H+] + [OH–] → [H3O+] + [OH–]
- In Pure Water [H3O+] = [OH–]
Much of the human body operates near pH7, which is said to be a neutral value. It is neutral because it is a safe amount of these ions for the structural protein molecules of the body. Proteins are large, charged molecules whose shape and function are modified by excess positive and negative ions in their environment. Ions physiology’s form of electricity.
Physiologic chemical electricity
Now that you know more about molecular energy and the chemistry of water, it is time to talk about the body’s form of electricity.
Physiology’s electricity is created by the flow of ions across cell membranes. Ions are atoms that have gained or lost an electron. In contrast, the electricity in your home is a flow of naked electrons through a wire.
Depending on an atom’s number of electrons, the ion it forms may have either a positive or a negative charge. There are four ions that are particularly important to explanations of physiology. They are sodium (Na+), potassium (K+), chloride (Cl–) and calcium (Ca++).
Ions diffusing through watery channels in cell membranes of the heart and brain create electrical currents. The charge of the ion blocks its ability to move through the cell’s lipid membranes. The membrane channels permit communication between the inside of cells and the extracellular milieu.
Cell membrane ion channels are made of large proteins. Proteins change their shape in response to changes in their environment. The shape changes cause opening and closing of the membrane ion channels. When channels are open electrical currents flow through the tissue.
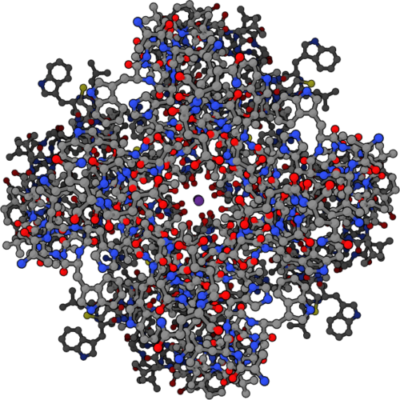
Four part symmetrical structure of potassium ion channel with potassium ion at center, Public Domain/Wikimedia Commons
Illustrated here is a molecular diagram of a K+ channel viewed from the inside of the cell. K+ is the purple dot in the center. Gray dots denote carbon atoms, blue dots hydrogen atoms and red dots oxygen atoms. Notice the circle of red, highly polar oxygen atoms surrounding the central purple dot.
Membrane channels are very selective about what they will let pass through. A Na+ cannot pass through a K+ channel and vice versa. Water molecules move through membrane channels designed specifically for water called aquaporins.
Ion flow through open membrane channels is driven by differences in the ion’s concentration on the two sides of the membrane as illustrated below.
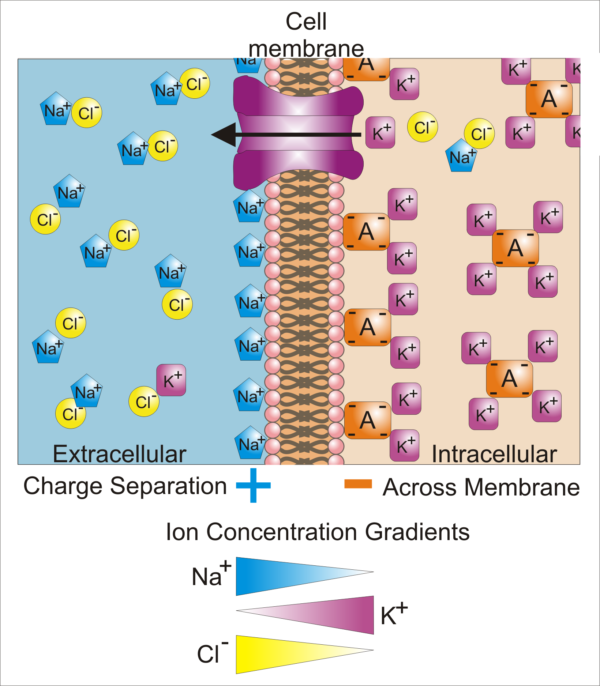
Difference in cellular ion concentrations and those of the extracellular fluid, Synaptidude/WikiMedia Commons
In this illustration there is a large open, purple K+ channel. Because the K+ concertation is higher inside the cell, K+ moves out of the cell when the potassium channel is open. In contrast, in this illustration, Cl– and Na+ remain where they are because there is no open channel pictured for them.
Chemical Pressures
There are several forms of chemical pressure that facilitate the movement of molecules through the body. They are osmotic pressure, hydrostatic pressure, and gas pressures.
Osmotic Pressure
The first of these, osmotic pressure is difficult for beginning students to understand. But it is also a very much used concept to explain delivery of nutrients by blood flow throughout the body.
As mentioned, water moves through biologic membranes if water channels, aquaporins, are present. The energy with which water molecules move through aquaporins is directly related to the amount of solute in the watery fluid on opposite sides of a membrane.
Notice in this illustration that the u-shaped tube on the left has different amounts of solute material on opposite sides of a water permeable membrane.
Over time, water will travel through the membrane to equalize the concentration of solute on both sides. This is what has happened in the u-tube on the right side of this illustration. Notice that the movement of water molecules through the aquaporins altered the height of the columns of water on opposites sides of the membrane.
Scientists used this experiment to define the energy driving water molecules through the aquaporins as a pressure. They showed that water could be prevented from leaving the side of the membrane with least solute if they added pressure to the side of the membrane with the greater concentration of solute.
The quantity of pressure needed in this experiment was equal to the weight of a column of water the height of delta H in the illustration. They named this quantity of pressure osmotic pressure.
What you need to remember about osmotic pressure is that it is higher for water solutions with a lot of solutes than for more dilute water solutions. Water molecules will move through aquaporins toward the side of the membrane with the highest concentration of solutes unless there is an opposing pressure.
Hydrostatic pressure
Hydrostatic pressure develops in fluids in a closed system when outside pressure is applied. Hydrostatic pressure increases in blood plasma with contraction of the heart’s ventricles.
At blood capillaries it is cardiovascular hydrostatic pressure that opposes the low solute concentration (low osmotic pressure) of the extracellular fluid. In the absence of blood’s hydrostatic pressure, water flows into rather than out of the blood capillaries.
The large arteries leaving the heart are stretched by blood forced into them. As blood flows away between heart beats, the elastic components of the large arteries recoil. Arterial recoil maintains hydrostatic pressure on blood plasma until the heart’s next contraction.
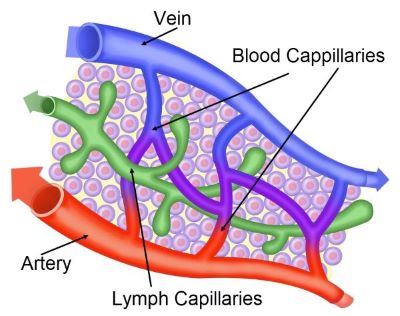
Both oxygen level and hydrostatic pressure decrease as blood moves through capillaries from artery to vein, Shutterstock.com
At the arterial end of a capillary bed, hydrostatic pressure opposes the osmotic pressure of the blood and water moves out of the blood vessels. Blood reaching the venous end of a capillary bed has lost most of its hydrostatic pressure. At the venous end, water lost at the atrial end is retrieved by the highly osmotic blood plasma.
Gas pressures
The mixture of atmospheric gas pressures is essential for proper function of the respiratory system. There are several chemical laws that describe the behavior of gas molecules.
One gas law is particularly important to explain how oxygen molecules enter the lung while carbon dioxide molecules move in the reverse direction. Its Name is Dalton’s Law of Partial Pressures.
In short, Dalton’s law says that each gas in a mixture will act as if the other gases are not there. It also states that each gas will move from an area where its concentration is high to an area where its concentration is low. Gas molecules, like other molecules, diffuse away from where their concentration is higher to where it is lower.
In this illustration, notice how low the gauge is for carbon dioxide [CO2] in air. The lungs’ gas is always higher in CO2 than air. So, CO2 diffuses out of the lungs.
In contrast, lung oxygen [O2] is always lower than in air. This is because capillary red blood cells grab oxygen entering the lungs and carry it to distant parts of the body. In this case, O2 flows into the lung’s gaseous mixture where its concentration is extremely low. The two gases O2 and CO2 move independently of each other.
Summary
To avoid struggling with chemistry and physiology, keep in mind these basic parts of chemistry:
- Chemistry’s definition of molecular energy is movement
- pH is a property of water solutions
- Physiologic electricity is carried by ions
- Diffusion of molecules proceeds from where their concentration is high to where their concentration is low
- Cell membranes have selective ion channels that are only open under certain conditions
- The f osmotic pressure of a water solution is directly related to the number of dissolved particles
- Hydrostatic pressure in a closed fluid system opposes the osmotic pressure of dissolved solutes in the fluid
- Atmospheric gasses act independently of each other
Further reading:
Read about how basic chemistry permits long distance communication between body parts in my book “Physiology: Custom-Designed Chemistry.” Click here for more information.
Do you have a plan for learning physiology?
If you would like to know how to apply the chemistry described above to learning Anatomy & Physiology check out my 30-day Challenge: Craft Your Plan for Learning Physiology. This online course of 30 short videos can be finished at your own pace. Try it before taking your next exam. by clicking here.
Margaret Thompson Reece PhD, physiologist, former Senior Scientist and Laboratory Director at academic medical centers in California, New York and Massachusetts is now Manager at Reece Biomedical Consulting LLC.
She taught physiology for over 30 years to undergraduate and graduate students, at two- and four-year colleges, in the classroom and in the research laboratory. Her books “Physiology: Custom-Designed Chemistry”, “Inside the Closed World of the Brain”, and her online course “30-Day Challenge: Craft Your Plan for Learning Physiology”, and “Busy Student’s Anatomy & Physiology Study Journal” are created for those planning a career in healthcare. More about her books is available at https://www.amazon.com/author/margaretreece. You may contact Dr. Reece at DrReece@MedicalScienceNavigator.com, or on LinkedIn.
Dr. Reece offers a free 30 minute “how-to-get-started” phone conference to students struggling with human anatomy and physiology. Schedule an appointment by email at DrReece@MedicalScienceNavigator.com.
Comments
Physiology: Why Learn Its Chemistry Now — No Comments
HTML tags allowed in your comment: <a href="" title=""> <abbr title=""> <acronym title=""> <b> <blockquote cite=""> <cite> <code> <del datetime=""> <em> <i> <q cite=""> <s> <strike> <strong>