Tips for How to Study the Cardiovascular System
Cardiac output: intrinsic, neural and endocrine regulation
Heart as a double pump
The cardiovascular system, the body’s pressurized blood re-circulation system is powered by a double pump, the heart. Cardiac output of the heart is regulated by intrinsic factors, the nervous system and the endocrine system. The human heart is composed of two filling and two pumping chambers, the atria and the ventricles. The right ventricle, a low-pressure output system drives blood into the thin walled arteries of the lung, the pulmonary arteries.
In the lung carbon dioxide, a waste product of metabolism is removed from blood and oxygen is added. Oxygenated blood returns to the left chambers of the heart through the pulmonary veins and is pumped by the left ventricle into the aorta with enough force to carry it to distant parts of the body.
Cardiac muscle
The model for building tension by cycling of actin and myosin cross bridges is the same for cardiac muscle and skeletal muscle. However, cardiac muscle is organized somewhat differently. Unlike straight-line skeletal muscle cells with their many nuclei per cell, cardiac muscle cells contain only one nucleus that is located centrally, and the cells are branched.
Myosin filaments are fewer and thicker in cardiac muscle than in skeletal muscle. Cardiac muscle has larger T-tubules than skeletal muscle. Cardiac T-tubules are highly branched mesh-like structures anchored to Z discs of myofibril sarcomeres. They are ducts of varying diameter composed of complex folded membranes.
Micro-domains within cardiac T-tubules position clusters of voltage-gated calcium ion channels near the sarcoplasmic reticulum’s ion channels. Ca++ entering through T-tubules during cardiac action potentials triggers release of Ca++ from the sarcoplasmic reticulum, which in turn initiates contraction of the sarcomeres.
Protein structures called intercalated discs tie cardiac muscle cells to each other creating a network. Ease of movement of ions and other material within the network is aided by the presence of gap junctions between cells at the intercalated discs.
There are two types of muscle cells in the heart, contracting muscle cells and conducting muscle cells. Contracting muscle cells make up most of the atria and ventricles and generate the force and pressure required to eject blood.
In the image 1. Sinoatrial (SA) Node; 2. Atrial Ventricular (AV) Node; 3. Bundle of His; 4. Left Bundle Branch; 5. Left Posterior Fascicle; 6. Left Anterior Fascicle; 7. Left Ventricle; 8. Ventricular Septum; 9. Right ventricle.
The conducting muscle cells, shown in blue in the image above, contribute little to the generation of force. Their function is to spread action potentials in a coordinated manner to trigger simultaneous muscle contraction over the entire heart.
Cardiac action potential
Contraction of cardiac muscle requires action potentials like skeletal muscle. But, in the heart cardiac action potentials originate in the pacemaker cells of conducting muscle rather than at a neuron synapse. Muscle cell action potentials use the same type of chemistry as neuron and skeletal muscle action potentials. A review of neuron action potentials can be found at “Neurons: Where Does Their Electricity Come From?”
There are some differences however between heart and skeletal muscle action potentials. Heart muscle action potentials last much longer, 150-300 milliseconds compared to 1-2 milliseconds for skeletal muscle. Cardiac action potentials display variable shape because of the participation of voltage-gated chloride [Cl–] and calcium [Ca++] channels in addition to the sodium [Na+] and potassium [K+] channels used by skeletal muscle and neurons.
The time course and shape of cardiac action potentials differs among ventricle, atrium, and pacemaker muscle. Yet, in all cases it is the amount of Ca++ entering during an action potential that governs the muscle force generated and the pace of heart pumping. This is because entering Ca++ is the trigger in heart muscle that releases stored calcium from the sarcoplasmic reticulum, which in turn initiates cross bridge formation between actin and myosin filaments and muscle shortening.
Cardiac pacemaker action potential
The primary pacemaker cells of the human heart are located at the sinoatrial [SA] node. Other conducting muscle cells with pacemaker capability exist along the tract of conducting muscle from the SA node to the tip of the ventricles. But because it is the fastest pacemaker, the SA node normally determines heart rate.
Inward flowing ions during a cardiac pacemaker action potential spread through muscle gap junctions to activate the tract of conducting muscle cells. Likewise, contracting muscle cells along the conducting tract are brought to threshold triggering their action potentials. The timing of the spread of action potential ions through the conducting tract and into contracting muscle brings large areas of contracting muscle to threshold simultaneously.
Frank Starling law of the heart
Pressure in the arteries depends upon the force of ventricular contraction and the amount of blood ejected. The Frank-Starling Law of the heart states that the volume of blood ejected by the ventricle depends upon the volume present at the end of the filling period (diastole). This relationship between stretch of heart muscle during filling and force of contraction ensures that the amount of blood ejected by the heart matches venous return to the heart.
This property of the heart is curiously different than what is observed with skeletal muscle. Stretching skeletal muscle weakens its force of contraction. Studies have established that during diastole cardiac sarcomeres are NOT optimally stretched. This allows cardiac muscle to adjust the length of its sarcomeres for optimal strength of contraction in response to a wide range of physiologic situations. In contrast, skeletal muscle is stretched to its best length for strong contraction by the fascia tying it to the skeleton.
But there is more to the Frank-Starling mechanism than initial length of the sarcomeres as the heart fills. There is also an increased sensitivity of the actin/myosin binding process to Ca++. One hypothesis is that stretching the sarcomeres increases the affinity of troponin for Ca++. An increase in troponin affinity for Ca++ would hasten the opening of myosin binding sites and speed up contraction of the muscle.
Blood pressure regulation
Blood is driven through the vascular system of arteries and veins by the difference in blood pressure between the arterial and venous sides of the circulation. Mean arterial pressure, the driving force behind blood flow is maintained at a set point of about 100 mm Hg [millimeters of mercury] by a continuously active neural feedback loop.
Baroreceptor reflex
Neural afferent sensory receptors for blood pressure, baroreceptors, are in the walls of the aortic arch and the carotid sinus. The carotid sinus is where the common carotid bifurcates into the internal carotid artery and the external carotid artery to the brain and face, respectively.
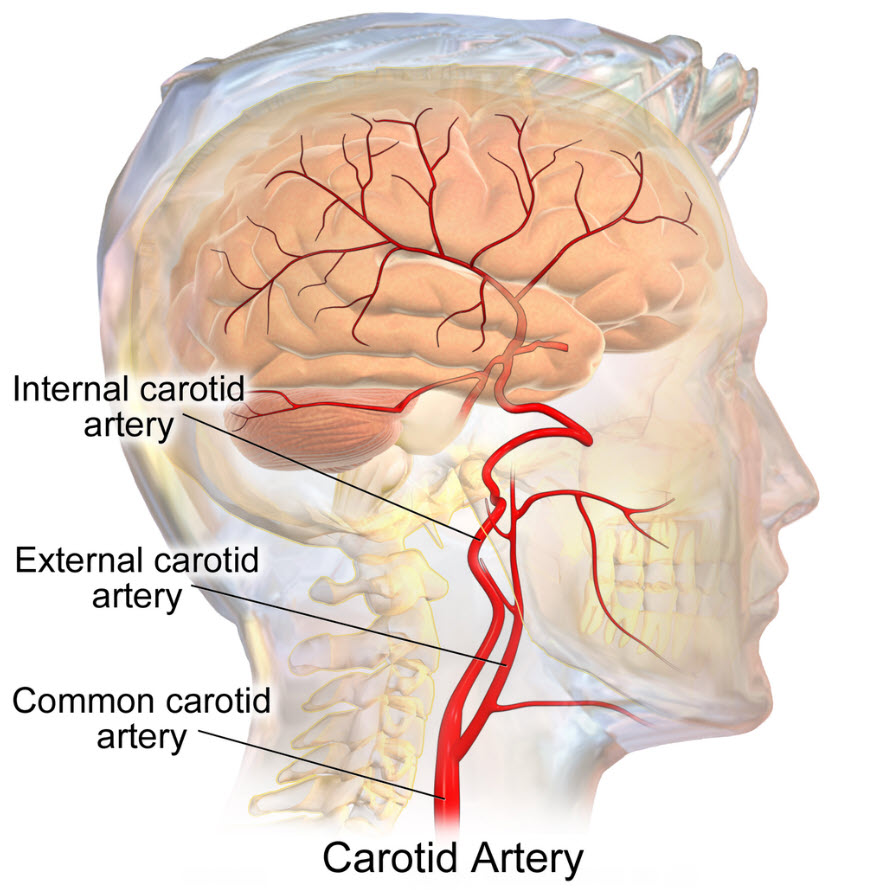
Baroreceptor location is at the bifurcation of the common carotid artery, Bruce Blausen/Wikimedia Commons
Baroreceptors are neuron afferents that respond to pressure and mechanical stretch of the arteries. They fire constantly and are particularly sensitive to the rate of change of arterial pressure. Their firing rate increases with increased arterial stretch and decreases with decreased pressure or arterial stretch.
Baroreceptor afferent signals travel to the brain stem in cranial nerve IX, the glossopharyngeal nerve (carotid sinus afferent), and cranial nerve X, the vagus nerve (aortic arch afferent). Their destination is the nucleus tractus solitarii in the brain stem which is also constantly active. This brain nucleus interprets changes in firing rates of the baroreceptor afferent and changes its own firing rate accordingly.
Autonomic control of heart
The nucleus tractus solitarii signals to the cardiovascular centers in the brain stem that control sympathetic and parasympathetic activity, the autonomic nervous system. Efferent sympathetic neurons synapse first in the spinal cord, then in the spinal ganglion and finally in the heart. Efferent parasympathetic neurons travel to the heart in the vagus nerve.
The sympathetic and parasympathetic brain centers work in a coordinated fashion to move blood pressure back to the mean arterial pressure set point of about 100 mmHg. Increased sympathetic activity, induced by low pressure in the large arteries, increases heart rate and contractility of cardiac muscle. It restricts blood flow to the surface arterioles of the vasculature and mobilizes increased venous return. The net result is increased cardiac output and a rise in pressure in the large arteries.
Parasympathetic firing of neurons in the vagus nerve, in response to high pressure in the large arteries, decreases heart rate and lessens the strength of contraction of cardiac muscle. A corresponding decrease in sympathetic activity opens blood flow at the peripheral arterioles. The net result is decreased cardiac output and a fall in pressure in the large arteries.
Blood volume regulation
Another component of maintaining an acceptable mean arterial pressure is preserving enough blood volume. Blood volume regulation requires a response of the endocrine system to supplement the reflex response of the neural baroreceptors.
Low blood volume
When blood volume is low there is insufficient venous return and decreased arterial pressure. To regain volume and pressure, the vasculature must increase its water and sodium [Na+] content. Increasing blood water and Na+ is accomplished by the kidney nephrons.
When the kidney senses that Na+ is too low in the filtrate flowing into the distal tubules of the nephrons, an indicator of low blood pressure due to low volume, it secretes a molecule named renin into the blood of the peritubular capillaries. Low filtrate Na+ indicates low blood volume because water follows Na+ due to osmotic pressure gradients created by Na+ molecules. If the blood concentration of Na+ is low, then the amount of water in the arteries is also low. Renin sets in motion the serial conversion of molecules in blood to form a hormone named angiotensin II.
Angiotensin II compensates for low blood volume in several ways. It vasoconstricts the arteries to increase blood pressure. It decreases glomerular filtration rate by constricting both the afferent and efferent arteriole. This allows more time for Na+ reabsorption in the tubules.
Angiotensin II enhances Na+, Cl– and water reabsorption by the proximal tubular cells for delivery to the peritubular capillaries. It stimulates vasopressin release from the posterior pituitary. Vasopressin increases membrane aquaporins so that H20 follows Na+ into the peritubular capillaries. Angiotensin II also causes release of the steroid hormone aldosterone from the adrenal glands which sit on top of the kidneys.
Aldosterone turns on gene transcription in the epithelial cells of the distal tubule and collecting duct for the proteins making up Na+ ion transporter complexes. Under the influence of aldosterone, the density of Na+ transporters in apical membrane of these cells increases 2- to 5- fold. Blood volume and blood pressure are returned to normal augmenting the sympathetic response of the large artery baroreceptors.
High blood volume
When blood expands to a greater than normal volume, pressure in the large arteries and in the atria of the heart rises. Large artery baroreceptors change their activity and the cardiovascular control centers in the brain stem respond. In addition, mechanical stretch of the wall of the atrium causes release of a hormone from cardiac muscle cells named atrial natriuretic peptide (ANP).
ANP travels to the kidney and increases the kidney’s blood filtration rate by altering the diameter of the glomerulus arterioles. With higher filtration pressure, more water and Na+ move into the nephrons’ tubules. Large amounts of Na+ in the distal tubules decreases renin secretion by the juxtaglomerular cells. ANP also directly inhibits synthesis of renin and aldosterone.

Juxtaglomerular apparatus near afferent arteriole of kidney glomerulus, OpenStax College/Wikimedia Commons
ANP stimulates protein kinases that inactivate the sodium ion transporters in the distal tubule and collecting duct. And, acting at the brain it suppresses salt appetite and vasopressin release.
ANP also augments the drop in sympathetic and increase in parasympathetic activity initiated by the baroreceptors of the large arteries in the presence of higher blood pressure. ANP accomplishes this by relaxing the smooth muscle of the arterioles and venules by inhibiting the norepinephrine released by the remaining basal activity of the sympathetic nervous system.
Although the heart is autonomous about regular pacing of its contractions and therefore cardiac output, it still depends greatly on fine tuning of its operation by the nervous, renal and endocrine systems. The interdependence of the nervous, cardiovascular and endocrine systems is a common theme in physiology.
Related articles:
Do you have questions?
Please put your questions in the comment box or send them to me by email at DrReece@MedicalScienceNavigator.com. I read and reply to all comments and email.
If you find this article useful, please share it with your fellow students. Click your favorite social media button.
Margaret Thompson Reece PhD, physiologist, former Senior Scientist and Laboratory Director at academic medical centers in California, New York and Massachusetts is now Manager at Reece Biomedical Consulting LLC.
She taught physiology for over 30 years to undergraduate and graduate students, at two- and four-year colleges, in the classroom and in the research laboratory. Her books “Physiology: Custom-Designed Chemistry”, “Inside the Closed World of the Brain”, and her online course “30-Day Challenge: Craft Your Plan for Learning Physiology”, and “Busy Student’s Anatomy & Physiology Study Journal” are created for those planning a career in healthcare. More about her books is available at https://www.amazon.com/author/margaretreece. You may contact Dr. Reece at DrReece@MedicalScienceNavigator.com, or on LinkedIn.
Dr. Reece offers a free 30 minute “how-to-get-started” phone conference to students struggling with human anatomy and physiology. Schedule an appointment by email at DrReece@MedicalScienceNavigator.com.
Comments
Tips for How to Study the Cardiovascular System — No Comments
HTML tags allowed in your comment: <a href="" title=""> <abbr title=""> <acronym title=""> <b> <blockquote cite=""> <cite> <code> <del datetime=""> <em> <i> <q cite=""> <s> <strike> <strong>