Body fluids and blood volume regulation
To maintain the appropriate composition of body fluids and to carry out blood volume regulation, the kidney works in tandem with the endocrine system. Shifting study of anatomy and physiology from bones and muscles to study of kidney physiology is a challenge, because the anatomy of the functional structure in a kidney, the nephron is only seen with a microscope.
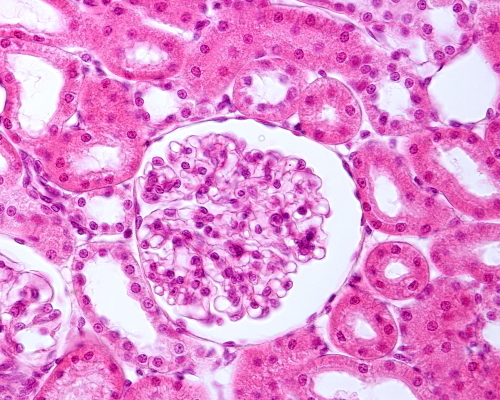
Histology section showing the kidney capsule, glomerulus and surrounding kidney tubules of a nephron, Jose Luis Calvo/Shutterstock.com
Learning how the kidney uses its microscopic structures to maintain a healthy composition of blood and interstitial fluid requires imagination and the use of cell models.
Kidney function
- Removal of water-soluble metabolic waste products from blood
- Regulation of the quantity of blood electrolytes, the water-soluble atoms carrying an electrical charge such a sodium [Na+] and potassium [K+] and phosphate [HPO42- ]
- Long term maintenance of blood pH [H+]
- Safeguarding of plasma osmolality, and thereby blood and interstitial fluid volume
While accomplishing this, a kidney must not lose any of the valuable water-soluble nutrients delivered by blood to tissues such as amino acids and glucose.
Urine formation
The kidney nephrons form urine to remove waste material and excess water from the body. Removal of water-soluble waste products of metabolism is accomplished by forcing part of the liquid portion of blood into a set of tubules, a process called filtration. It then returns to the blood from the tubules those molecules that are important to keep like water, amino acids, glucose, Na+ and K+, a process called resorption. The portion of water, excess molecules and waste that is not reabsorbed into blood exits the kidney as urine and is delivered to the bladder by tubes called ureters.
Large waste molecules in blood that are not filtered easily by the nephrons are transported into the tubules by another process called secretion. The walls of the kidney tubules, in cooperation with proteins in the walls of the surrounding blood vessels transfer large waste molecules into the kidney tubule lumen for elimination from the body with urine.
It is extremely important to body tissue that the quantity of particular charged particles remains at near a constant level in blood and in the protein-free interstitial fluid surrounding cells. For example, both heart muscle contraction and neuron signaling require precise interstitial fluid levels of Na+ and K+ and Ca++. The concentration of these ions in interstitial fluid depends to a large extent upon their concentration in blood.
Steady, long-term regulation of blood pH also occurs at the kidney. Excess hydrogen ion [H+], that is H+ not buffered by bicarbonate and other blood buffers, is secreted from blood into the tubules where it complexes with positively charged ammonium. Ammonium is synthesized by the cells of the tubules and secreted into their lumen to capture the free H+ and eliminate it in urine.
Kidney blood flow
The arteries to the kidney, the right and left renal arteries, branch directly from the abdominal aorta. The renal artery divides as it enters the kidney into segmental arteries.
The segmental arteries continue to divide as they move deeper toward the renal cortex which lies just under the kidney’s fibrous capsule. In the renal cortex, the arterial branches form the arterioles of the kidney’s blood filtration unit, the glomerulus.
Although not shown in the above image, nerves of the sympathetic and parasympathetic system also travel through the kidney in close association with the arteries.
Kidney nephron
The functional unit of a kidney is named a nephron. There are approximately one million nephrons in the cortex of each human kidney. Nephrons consist of a capsule containing an elaborate cluster of specialized blood capillaries named Bowman’s capsule and a set of tubular structures.
The tubular structures are surrounded by a capillary network that leads to venules. Venules merge to form the veins leaving the kidney. Kidney veins follow the path of the incoming arteries, but in a reverse direction. Venous blood exiting the kidney flows into the inferior vena cava for return to the heart.
The illustration above suggests more open space between the tubules and capillaries than exists in a kidney. Check out the photomicrograph at the beginning of this article and notice how closely the tubules pack around the glomerular capsule allowing only small spaces for the capillaries.
Glomerular filtration
The first step in processing of blood in the kidney is accomplished by the glomerulus, the cluster of capillaries within the nephron’s Bowman’s capsule.

Juxtaglomerular apparatus near afferent arteriole of kidney glomerulus, OpenStax College/Wikimedia Commons
Blood capillaries of Bowman’s capsule are different than capillaries elsewhere in the body. Other blood capillaries spread out in a net-like pattern with an arteriole at one end feeding blood to them and a soft-walled vessel named a venule at the other end draining blood back toward the heart.
In contrast, the cluster of capillaries in Bowman’s capsule has an arteriole on both ends. This is important because it allows blood pressure within the glomerular capillaries, and therefore the rate of filtration, to be fine-tuned by changing the diameter of either the afferent arteriole (incoming blood) or efferent arteriole (exiting blood).
Capillaries of Bowman’s capsule possess numerous large open holes called fenestrae. The fenestrated capillaries are covered by cells with foot-like projections named podocytes. The foot processes of podocytes form slit-like barriers over the fenestrae that limit the amount of larger molecules such as protein that can be filtered into the lumen of Bowman’s capsule and on to the proximal convoluted tubule.
Kidney autoregulation
The rate at which blood constituents filter into kidney tubules is determined by blood pressure in the glomerular capillaries. The kidney has multiple mechanisms for autoregulation of glomerular blood pressure. But the primary mechanism is the response of the afferent arteriole to changes in overall blood pressure in the body, systemic blood pressure.
The wall of arterioles contains smooth muscle cells. The response of these cells to stretch is to shorten their length. High systemic blood pressure stretches smooth muscle of the arteriole, the muscle contracts and the diameter of the arteriole becomes smaller permitting less blood to flow through. The reverse happens when systemic blood pressure is low. As systemic blood pressure drops, the muscle cells of the arteriole relax and blood flow increases.
Blood volume regulation
Variation in blood volume affects blood pressure. Maintaining an optimal blood volume set point is another function of the kidney. Blood volume is regulated by Na+ and water reabsorption by the kidney. The Na+ concentration in the glomerular filtrate is monitored by cells in the distal tubule called the macula densa.
Low blood volume
The macula densa cells are in the part of the distal tubule near the collecting duct that travels between the afferent and efferent arteriole. If there at too few Na+ in the filtrate indicating low blood volume, the macula densa cells signal to the cells of the juxtaglomerular apparatus (refer to the illustration above) to release a hormone named renin into blood of the efferent arteriole.
Renin acts as an enzyme to convert another blood molecule from its inactive form to its functional form. Renin converts angiotensinogen which is continuously secreted by the liver to angiotensin I. Angiotensin I is then converted to angiotensin II by the angiotensin converting enzyme (ACE1).
Angiotensin II compensates for low blood volume in several ways. It vasoconstricts the arteries to increase blood pressure. It decreases glomerular filtration rate by constricting both the afferent and efferent arteriole. This allows more time for Na+ reabsorption in the tubules. It enhances Na+, Cl– and water reabsorption by the proximal tubular cells for delivery to the peritubular capillaries. It stimulates vasopressin release from the posterior pituitary. Vasopressin increases membrane aquaporins so that H20 follows Na+ into the peritubular capillaries.
Angiotensin II also causes release of the steroid hormone aldosterone from the adrenal glands which sit on top of the kidneys. Aldosterone turns on gene transcription in the epithelial cells of the distal tubule and collecting duct for the proteins making up Na+ ion transporter complexes. Under the influence of aldosterone, the density of Na+ transporters in apical membrane of these cells increases 2- to 5- fold.
High blood volume
High blood volume stretches the walls of the atria. Excess stretch of the atrial myocardium triggers release of peptide hormones called atrial natriuretic peptide (ANP).
ANP inhibits the renin, angiotensin II, aldosterone hormone system that increases Na+ and H2O reabsorption by reducing synthesis and release of renin and aldosterone. It also stimulates protein kinases that inactivate the sodium ion transporters of the distal tubule and collecting duct.
ANP increases glomerular filtration rate by dilating the afferent arteriole while maintaining constriction of the efferent arteriole. And, acting at the brain it suppresses salt appetite and vasopressin release.
ANP also augments the drop in sympathetic and increase in parasympathetic activity initiated by the baroreceptors of the large arteries in the presence of higher blood pressure. ANP accomplishes this by relaxing the smooth muscle of the arterioles and venules by inhibiting the norepinephrine released by the remaining basal activity of the sympathetic nervous system.
In summary
Low blood volume initiates a process facilitating Na+ and H2O reabsorption by the capillaries of the kidney nephrons. This is a physiologic set point regulation that brings together activity of the cardiovascular, endocrine, and nervous systems.
High blood volume initiates atrial secretion of ANP, which inhibits the kidney’s reaction to low blood volume.
Do you have questions?
If you want to know more about how the kidney works, please put your questions in the comment box or send me an email at DrReece@MedicalScienceNavigator.com. I read and reply to all comments and email.
If you find this article helpful share it with your fellow students or send it to your favorite social media by clicking on one of the buttons below.
Further reading:
Hormone Regulation of Plasma Calcium: Human
Tips for How to Study the Cardiovascular System
3 Simple Secrets to Learning Physiology
Do you have questions?
Send me an email with your questions at DrReece@MedicalScienceNavigator.com or put them in the comment box. I always read my mail and answer it. Please share this article with your fellow students taking anatomy and physiology by clicking your favorite social media button.
Margaret Thompson Reece PhD, physiologist, former Senior Scientist and Laboratory Director at academic medical centers in California, New York and Massachusetts is now Manager at Reece Biomedical Consulting LLC.
She taught physiology for over 30 years to undergraduate and graduate students, at two- and four-year colleges, in the classroom and in the research laboratory. Her books “Physiology: Custom-Designed Chemistry”, “Inside the Closed World of the Brain”, and her online course “30-Day Challenge: Craft Your Plan for Learning Physiology”, and “Busy Student’s Anatomy & Physiology Study Journal” are created for those planning a career in healthcare. More about her books is available at https://www.amazon.com/author/margaretreece. You may contact Dr. Reece at DrReece@MedicalScienceNavigator.com, or on LinkedIn.
Dr. Reece offers a free 30 minute “how-to-get-started” phone conference to students struggling with human anatomy and physiology. Schedule an appointment by email at DrReece@MedicalScienceNavigator.com.